The previous post was about an insecticide and made a point that the persistence of both insecticides and herbicides is an important aspect of their environmental properties. Water hydrolysis will degrade them, a typical residency time being in the order of a few days. I noted in passing a dioxepin-based herbicide[cite]10.1039/P29890001265[/cite] which contains a ketal motif and which in water can hydrolise to a ketone and alcohol. The reverse (acid catalysed) formation of a ketal is a staple of the taught organic chemistry curriculum. Here as a prelude to looking at the hydrolysis of that dioxepin, I take a look at a possible computational mechanism for the hydrolysis of 2,2-dimethoxypropane using pure water, without the help of acid or base.
The model includes up to three water molecules acting as proton transfer agents in eight-membered ring cyclic transition states (ωB97XD/Def2-SVP/SCRF=water, FAIR DOI: 10.14469/hpc/8043). One point of interest is the anomeric effects that are set up in the various species.
The proposed model for the mechanism is shown above, involving elimination/re-addition at a C-C bond.
Species | Relative energy kcal/mol, Def2-SVP |
Relative energy, |
DOI |
---|---|---|---|
Ketal+3H2O | 0.0 | 0.0 | 8042,8054 |
TS1: ketal to enol | 40.6 | 36.8 | 8037,8053 |
Enol | 16.7 | – | 8039 |
TS2: Enol to hemiketal | 42.8 | 38.6 | 8032,8050 |
Hemiketal | 1.7 | 5.0 | 8049,8052 |
TS3: hemiketal to ketone | 22.8 → 18.6 | 28.4 → | 8030,8051 |
Ketone+2MeOH+2H2O | 1.0 | – | 8041 |
The initial ketal species is shown below. Note particularly how the oxygen about to become protonated shows a longer O-C bond than that remaining in situ. This is because of how the lone pairs on oxygen align with the adjacent C-O bond, the “anomeric effect”. It predisposes one of the methoxy groups to removal by this partial weakening of the bond. The effect is also seen later in the mechanism for the hemiketal, with the methoxy group having the longer C-O bond, again preparing it for removal by protonation via TS3 rather than the hydroxy group.
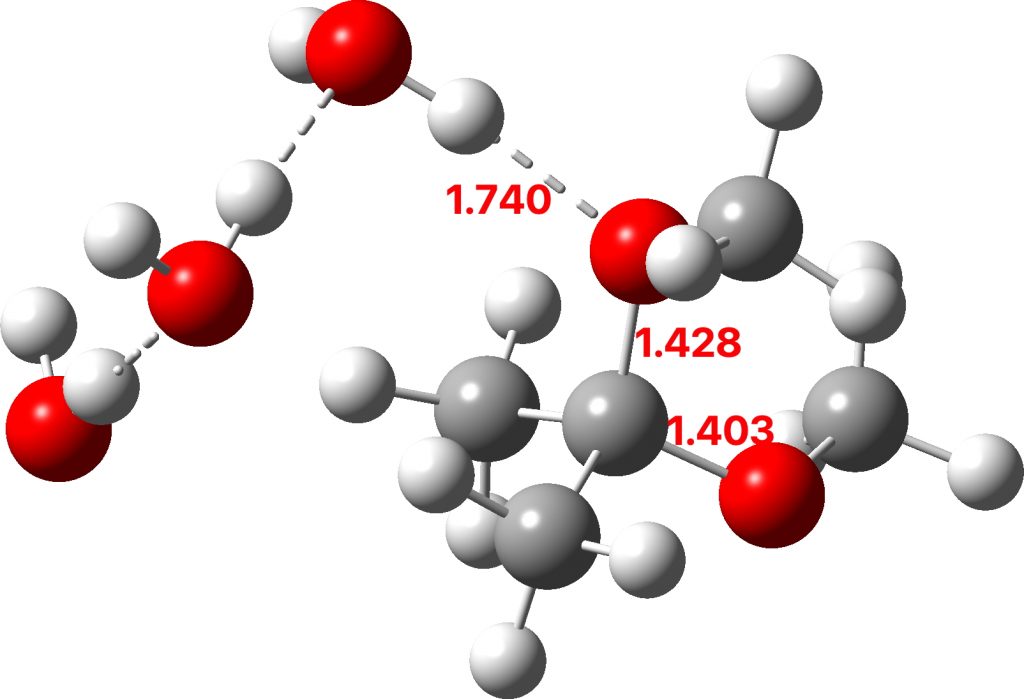
Reactant. Click to load 3D model
The TS1 that results from this protonation shows a relatively high free energy barrier of ~41 kcal/mol and the typical flat region of the transition state typical of highly ionic mechanisms.
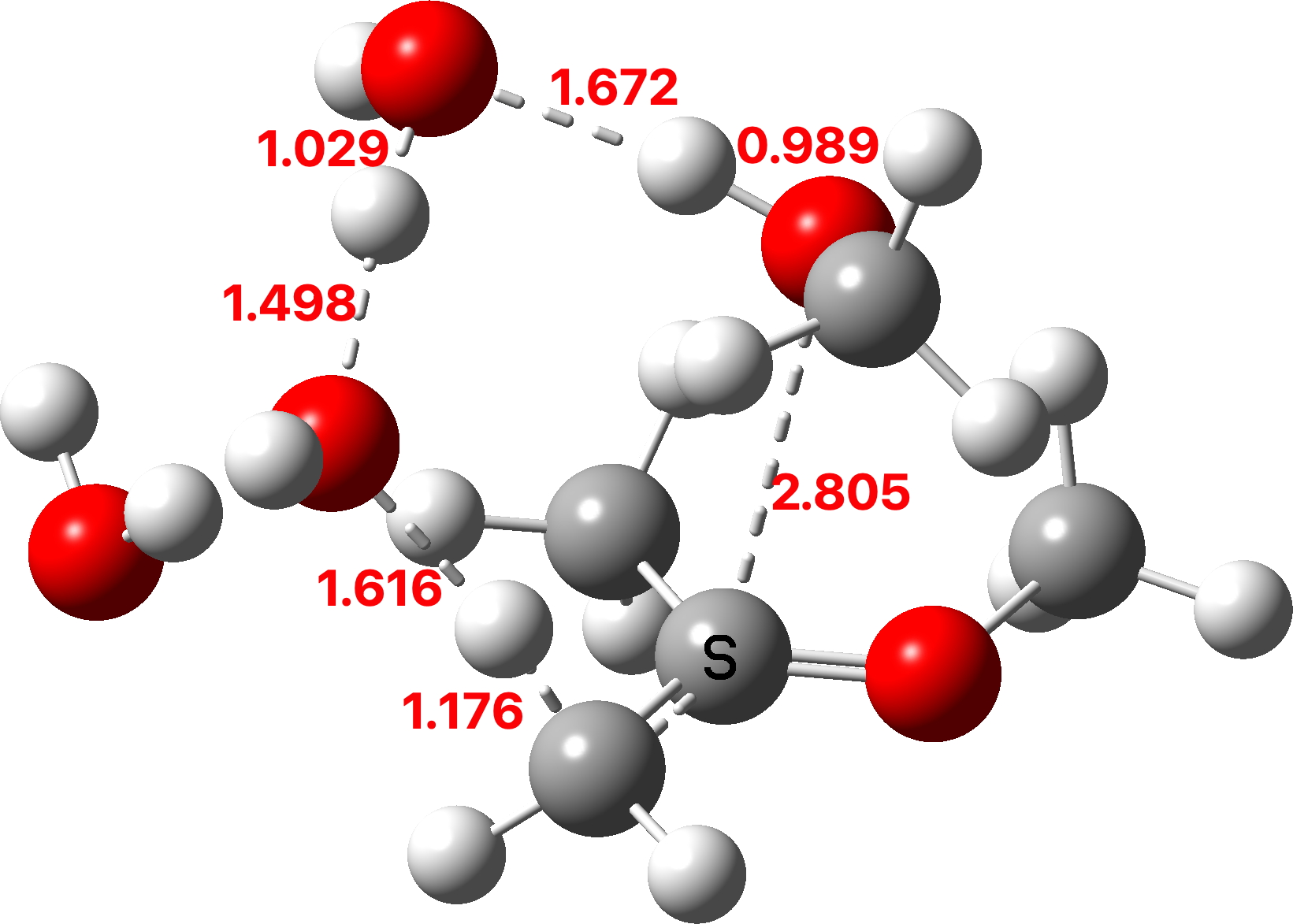
TS1: Click to view 3D model
The first part of the mechanism involves a late proton transfer from water to the oxygen of one methyl group at the transition state with departure of methanol, to form an oxenium cation-like structure with solvated hydroxide anion as the counter ion. Then in the second phase of the reaction, the hydroxide anion removes the hydrogen from a methyl group to form the first intermediate, an enol and methanol. The reaction is however concerted, albeit highly asynchronous.
Next, a water donates a proton to the sp2 carbon of the enol to reform a methyl group to form a temporary oxenium cation/hydroxide anion ion pair. Finally a water molecule attacks at the carbon of the oxenium cation to collapse the ion pair by one more proton transfer back to the hydroxide anion to form a hemiketal. The overall effect of these two steps is to perform in effect an SN2 substitution, with water replacing methanol.
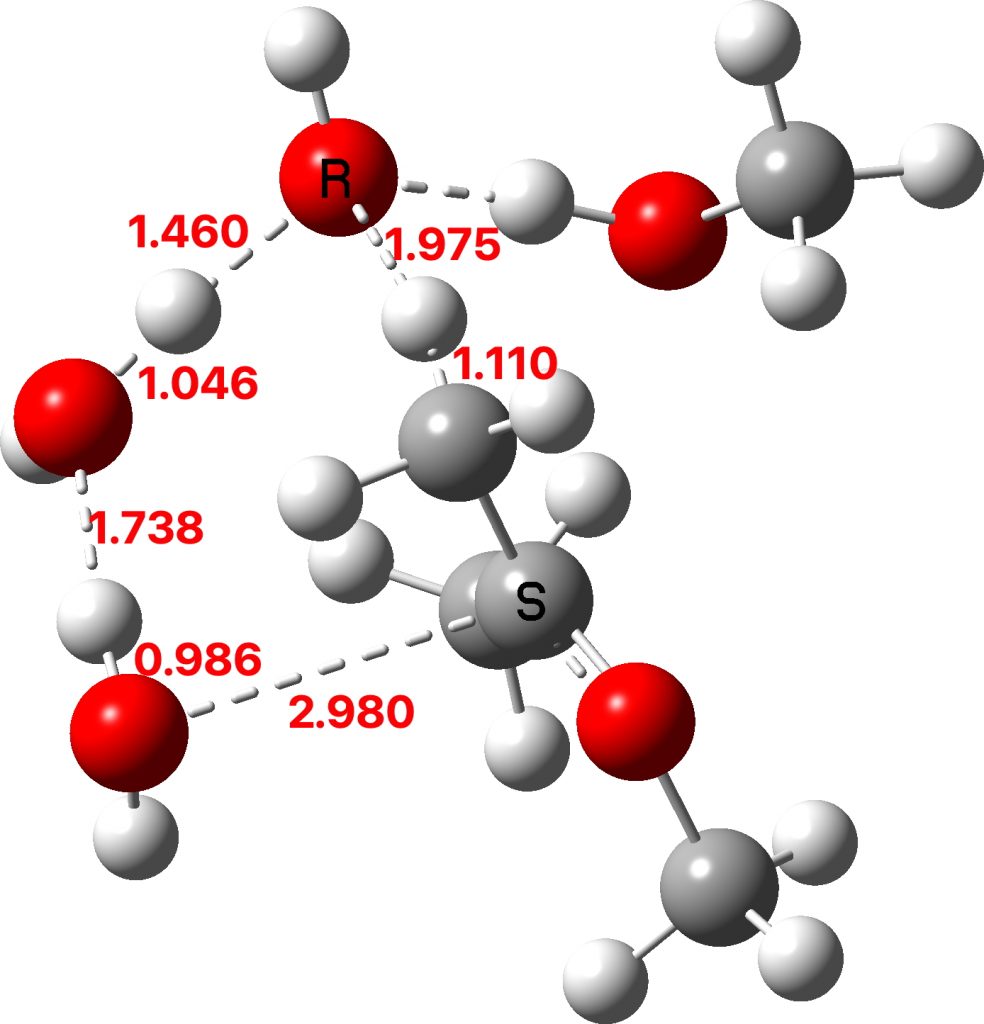
TS2: click to view 3D model
The hemiketal formed shows a very strong asymmetric anomeric effect, in which the remaining C-OMe bond is already strongly pre-disposed to cleavage.
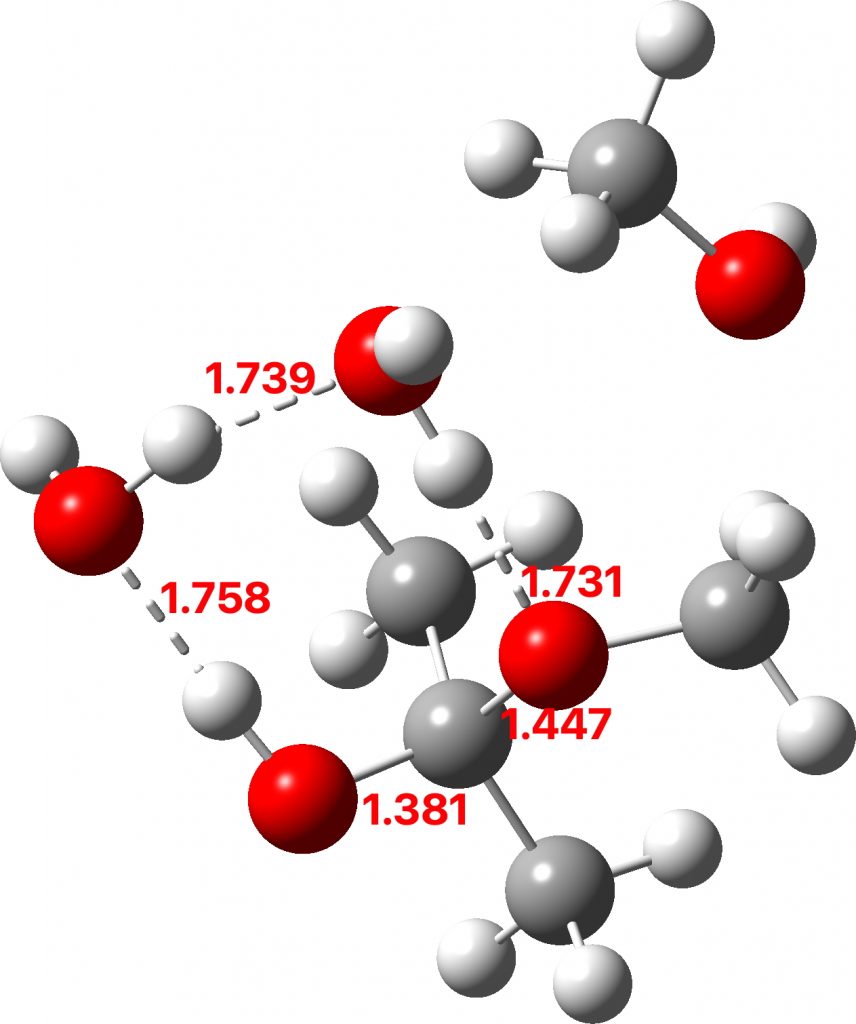
Hemiketal: Click to view 3D model
To complete the reaction, water abstracts the proton from the hemiketal OH group and via a relay places another proton on the oxygen on the remaining methoxy group, causing it to cleave the C-O bond. Result: ketone + two methanols + two catalytic waters as the end product.
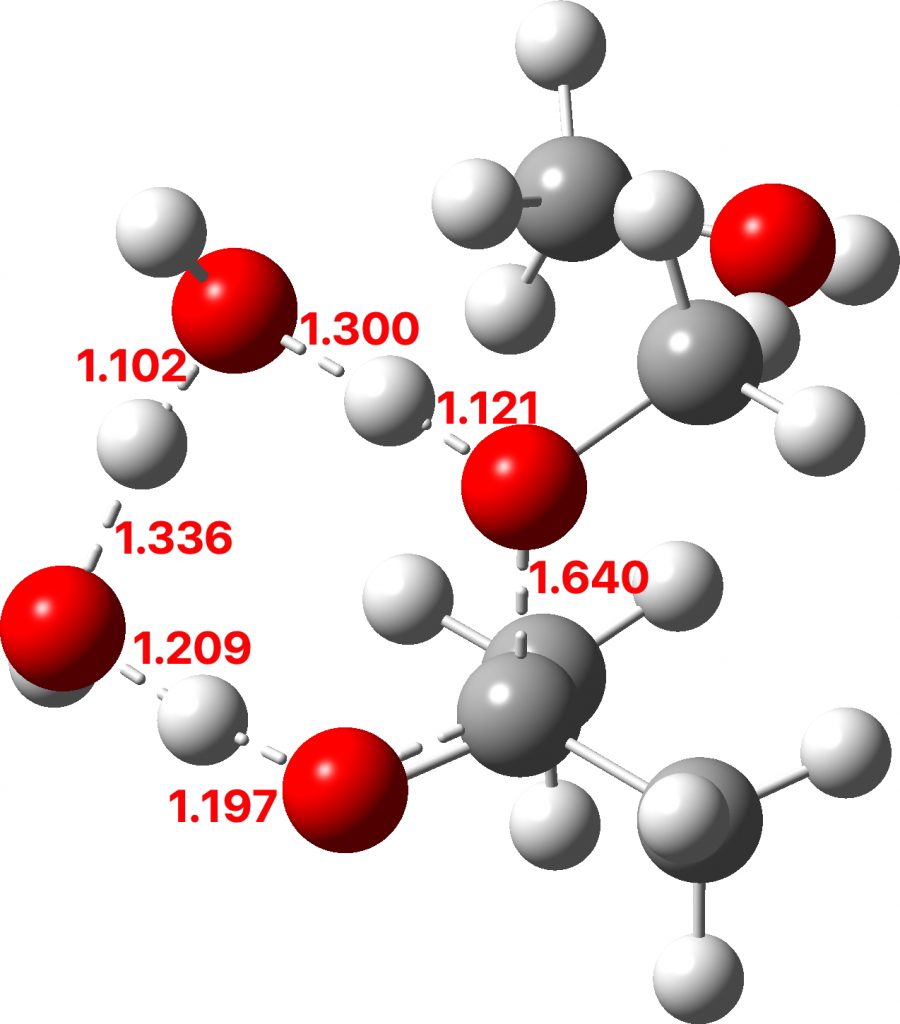
TS3: Click to view 3D model
Armed with a model for the aqueous hydrolysis of a ketal, one can now apply this model to isomeric ketals, one of which is known to hydrolize faster than the other, to see if this model can replicate the observation. One must also be aware that it will never be easy to disentangle the rate of hydrolysis by “pure water”, ie H2O, from the rate of acid or base catalysed hydrolysis. Recollect that even in “pure water”, autoionisation means that [OH–] and [H3O+] are both 10-7M and so both these species could also contribute to the catalytic rate. The ratio of [H2O]/[OH–] in water corresponds to a free energy difference of 11.9 kcal/mol. So if the free energy barrier to either the hydroxide or hydronium ion catalysis is at least 11.9 kcal/mol lower that of the free water energy barrier, the predominant hydrolytic contribution will be from these ionic species and not from water itself. With these thoughts in mind, watch this space!
Since this post is about more than one reaction beginning from the same starting point I’ll raise this here. Have you seen the article on hybrid light-matter states in the May 2021 issue of Physics Today? They describe the first steps towards a device that could allow the operator to choose which product they want out of a given set of starting materials, and describe a useful example. It sounds like being able to dial in, for instance, which product you want the above hydrolysis to make – ketone or enol ether.