The world’s smallest nano car was recently driven a distance of 6nm along a copper track. When I saw this, I thought it might be interesting to go under the hood and try to explain what makes its engine tick and its fuel work.
The molecule above represents (I think) the essentials of the engine. Its resting geometry in the S0 electronic state is shown below.
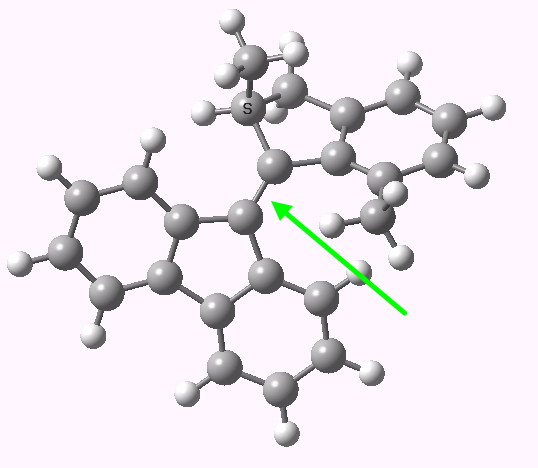
The resting geometry of the engine. The green arrow points to the axle, currently planar. Click for 3D model.
- Its journey is started by irradiation with light. This has the effect of injecting around 58.6 kcal/mol worth of “fuel” into the molecule, enough to excite the π-electrons in the axle (green arrow above) into a biradical excited S1 state, in the process turning the double into a single bond and hence a properly rotatable axle.
The minimum energy shape is different from the S0 ground state, involving a ~90° rotation around the axle. The crucial part here is that the axle is freely rotatable ONLY in a clockwise direction. In the anti direction, the benzo group on the right is prevented from moving up by a bump into the alkyl group above it. The energy profile for the clockwise rotation is shown below as downhill all the way, the driving force being a 13 kcal/mol drop in energy (calculated as a triplet rather than singlet state, ωB97XD/6-31G(d).
- The rotation passes the point of no return, so that when the short-lived excited S1 state decays back to the S0 state (via a conical intersection), the now restored C=C double bond finds it easier to continue the clockwise rotation a further ~60° (making 139° in total thus far), rather than to reverse its motion back to its original starting point. It is probably helped in this by its momentum as it emerges from the conical intersection. This new pose is 4.1 kcal/mol higher in free energy than the original starting one, a rather poor fuel economy for a half rotation of the wheel, given the 59 kcal/mol injection at the start!
The next resting position for the engine. Click for 3D.
- Back on the S0 state, the now un-rotatable double-bond axle acts as a ratchet mechanism, meaning that the next stage in the rotation of the wheel must occur elsewhere. This involves persuading the two groups indicated with a green arrow above to rotate past each other. At this stage the alkyl group is located underneath the benzo group, and so the latter has to rotate clockwise (and not anticlockwise) to achieve this.
- This step is accomplished with help from the tip of the STM microscope. When a high voltage is applied to the tip, electrons pass from the tip to the copper atoms on the surface, and experience what is called inelastic scattering as they tunnel through the molecule. As they do so, they lose energy to the molecule, which is presumed to pump up specific vibrations to help the molecule surmount what is calculated to be a 20.0 kcal/mol free energy barrier to rotation (the molecule has little vibrational thermal energy of its own since the experiment is conducted at low temperatures). If this barrier were much higher, it it might be that no amount of such vibrational excitation could induce the molecule to surmount the barrier.
- The experiments indicate a vibration of around 1600 cm-1 is excited, and the suggestion is that the axle C=C stretch is excited.
- However, I cannot help feeling that this might simply undo the molecular ratchet, and lose the accumulated clockwise specificity of the wheel rotation.
- A vibration is surely needed which would help the benzo group slip past the alkyl group, thus helping preserve the sense of direction. The calculated transition mode (click below) shows a fair degree of motion by the alkyl (methyl) group, as it contrives to avoid the benzo group.
- Clearly, these are questions that need investigation. Thus deuterating parts of the molecule (say the alkyl group) to see if rolling is inhibited might be an interesting experiment?
The rotational transition state. Click for 3D.
- Once past this barrier, the wheel has completed a total of 180° worth of rotation since starting, in two stages of 139 and 41°.
Two pairs of light pulses + vibrational excitations are required to make the wheel complete one whole rotation. But it is the mechanism whereby the light and the vibration co-operate to roll the vehicle forwards is what I find fascinating. What has not been addressed in the above analysis (of a single wheel) is how all four wheels cooperate to move the car forward. Does the light excitation rotate each wheel axle separately or simultaneously, thus involving one or four photons? Does the inelastic scattering due to electron tunneling excite just a single in-phase vibration of all four wheels? There are still mysteries (at least to me) for which I continue to seek an explanation.
Tags: conformational analysis, nanocar
[…] Henry Rzepa Chemistry with a twist « Under the hood of a nano car: the chemistry behind a molecular motor. […]
Ben Feringa, the principle author of the article above, is one of the recipients of the 2016 Nobel prize for chemistry. See http://www.kva.se/en/pressroom/2016/the-nobel-prize-in-chemistry-2016/