Introductory organic chemistry invariably features the mechanism of haloalkane solvolysis, and introduces both the Sn1 two-step mechanism, and the Sn2 one step mechanism to students. They are taught to balance electronic effects (the stabilization of carbocations) against steric effects in order to predict which mechanism prevails. It was whilst preparing a tutorial on this topic that I came across what was described as the special case of neopentyl bromide, the bimolecular solvolysis of which has been identified (DOI: 10.1021/ja01182a117) as being as much as 3 million times slower than methyl bromide. This is attributed to a very strong steric effect on the reaction, greater even than that which might be experienced by t-butyl bromide! Time I thought, to take a look at what might make neopentyl bromide so special, and what those supposed electronic and steric effects were really up to.
How does one construct a quantitative model? Well, a method which incorporates both van der Waals effects (dispersion attractions) and solvation in computing a potential energy surface seems appropriate. I used ωB97XD/6-311G(d,p) with SCRF correction for methanol as solvent. This predicts the following transition state structure. The calculated (free energy) barrier from the reactant is 30.2 kcal/mol.
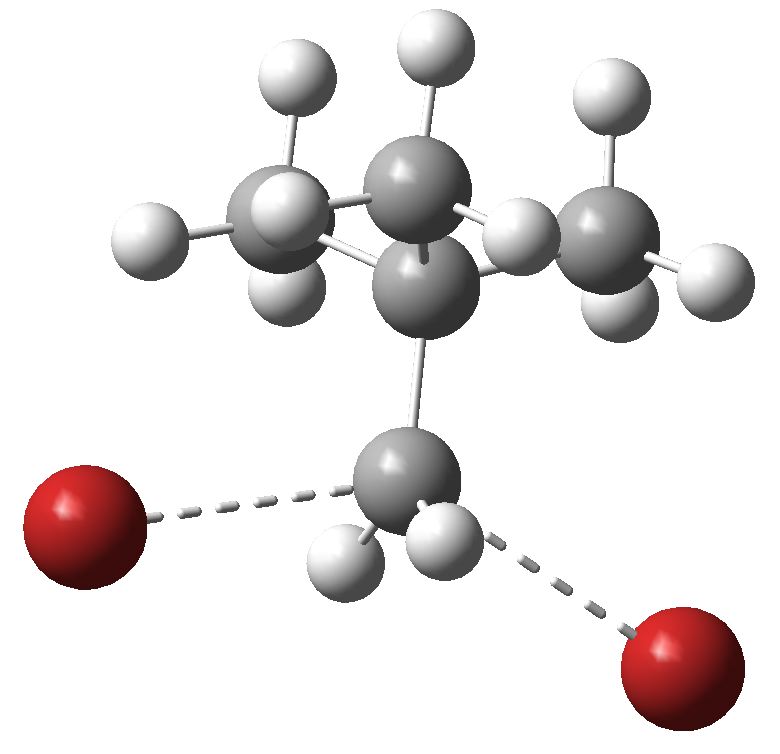
Sn2 transition state structure for neopentyl bromide. Click for 3D.
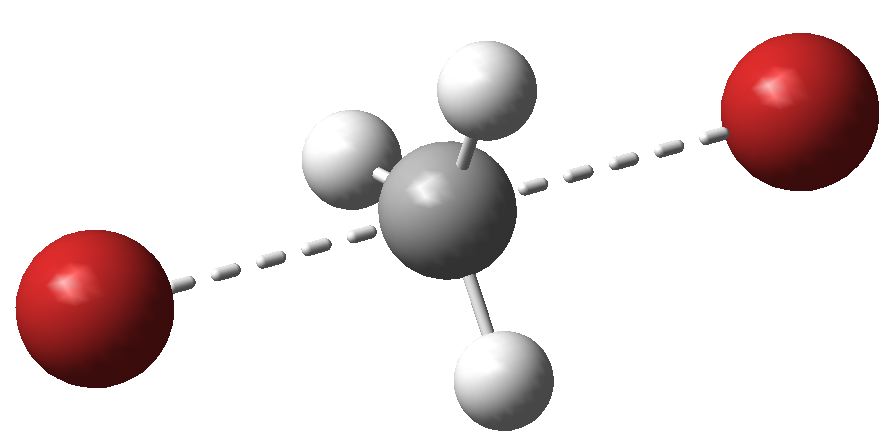
Sn2 transition state for methyl bromide. Click for 3D.
- Firstly, the difference in free energy of activation, ΔΔG, for the two reactions is 9.4 kcal/mol. We can apply this equation: ΔΔG = -RT ln (kmethyl/kneopentyl). This comes out at around 8.3 million (at 298K). The agreement with experiment is not at all bad (in reality, one might expect a better model to include explicit hydrogen bonding from the reagants to the solvent, which are neglected in this simple model).
- Next, notice that whilst the transition state for methyl bromide can sustain a linear arrangement of the Br…C…Br atoms, that for neopentyl bromide is quite bent, at 140° Why is it bent? To cast light on that, we need to know the van der Waals radii for H (1.1) and Br (1.95Å), giving a sum of 3.05Å. Any contact between these two that is significantly shorter than this value could be reasonably defined as steric bumps. Indeed, inspecting the model throws up four Br…H contacts of ~2.9Å. If the Br…C…Br atoms were linearly arranged, these bumps would be far worse. So neopentyl bromide is indeed sterically hindered!
- But wait, how about measuring the C…Br distances in the transition states? About 2.7Å for neopentyl, but noticeably shorter at 2.5Å for methyl bromide. We can interpret that as indicating that the neopentyl bromide transition state has a little more carbocation character than methyl bromide. The extreme manifestation of that would be an ion pair, or more accurately an ion triple, such as Br(-)…C(+)…Br(-). So, as the carbocation character increases, the steric effects would decrease!
- But this needs further calibration. So how about t-butyl bromide. Students of course are told that for this species, there is a change in mechanism from Sn2 to Sn1. What does the computer say? The calculated structure is shown below, and it reveals a barrier of 18.9 kcal/mol, with a C-Br length of 3.43Å. Well, the barrier is even lower than methyl bromide! And the C-Br distances almost 1Å longer. This supports the Br(-)…C(+)…Br(-) model, and confirms that lengthening the C-Br distance in the transition state does tend to indicate more ionic (i.e. Sn1) character. If you inspect the transition state vibrational mode, you will find another spectacular difference. For the Sn2 reaction proper, the motion is of mostly the two bromines and the central carbon. With the Sn1 mechanism, it is rotation of a methyl group (which is attempting to align with the carbocation centre being formed). This methyl motion is why such Sn1 reactions exhibit large secondary deuterium kinetic isotope effects! Again, the closest approaches between H and Br are ~ 2.9 -3.0Å, in this case very similar to the Sn2 mechanism.
Sn1 (Sn2) transition state for t-butyl bromide solvolysis. Click for 3D
So in this simple example, we can see illustrated many interesting effects; the balance between Sn1 and Sn2, the close approaches between atom pairs that characterize steric effects, the difference between fully ionic and less ionic structures, how the reaction normal mode (coordinate) changes with this changing mechanism. The text books have not gotten it wrong, but its nice to have some numbers associated with these concepts.
Tags: free energy, free energy barrier, Historical, potential energy surface, Tutorial material
[…] a second stage reacts with nucleophile (water) to form e.g. t-butanol. This is contrasted with the SN2 mechanism, where these two stages are conflated into a single concerted process, involving no intermediates. […]